Imaging and Image Guidance for Precision Small Animal Radiotherapy
The overall goal of this research is to establish advanced diagnostic imaging technologies and understand radiation-induced normal tissue toxicities. This group seeks to improve the understanding, diagnosis, and treatment of cancer through preclinical and clinical investigations, as well as the translation of novel forms of cancer imaging, radiobiologic agents, and precision radiotherapy.
Members
.jpg)
Amit Sawant, PhD (he/him)
Vice Chair of Medical Physics
Professor
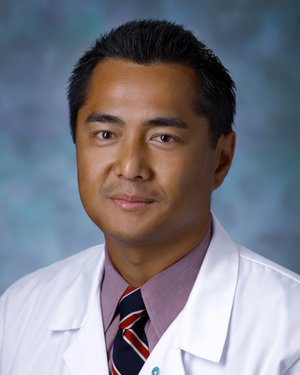
Phuoc Tran, PhD
Vice Chair of Research
Professor
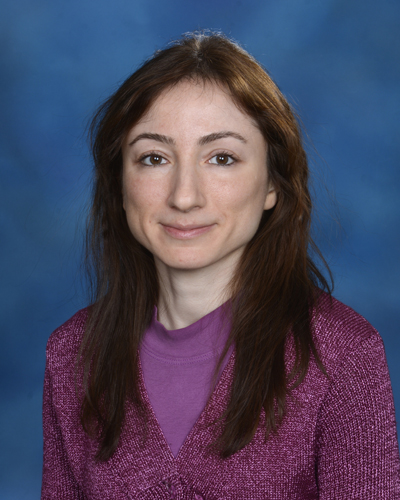
Arezoo Modiri, PhD
Assistant Professor
.jpg)
Kai Jiang, PhD
Assistant Professor
Major Research Areas
Small Animal Myocardial Functional MRI
Radiation-induced myocardial toxicity is a major limiting factor in treating centrally located thoracic tumors. Myocardial remodeling and functional alterations following radiation injury remains elusive. In addition, FLASH radiotherapy has lately been shown to offer sparing effects to normal tissues, but such effects remain to be investigated in the heart. To understand radiation-induced functional changes in the heart, an advanced rodent myocardial functional MRI technique has been established on a horizontal preclinical 9.4-T Bruker MRI scanner (Bruker Biospin Co., Billerica, MA). This technique allows measurement of both global and regional left ventricular function in rodents. Cine-MRI is used to capture a video of the beating heart for assessment of cardiac anatomy and global functional parameters such as ejection fraction and cardiac output. Displacement-encoding with stimulated echo (DENSE) MRI is used to assess regional cardiac function including the myocardial strain, ventricular twist, and torsion, parameters that have been shown to be more sensitive biomarkers of early functional changes than global functional parameters. Our major areas of research include:
- Understanding radiation-induced cardiac functional changes in our established mouse model of radiation-induced normal pulmonary injuries post bilateral lung irradiation;
- Investigation of the FLASH sparing effect on cardiac function using a rodent model of radiation-induced cardiotoxicity
Small Animal Image-Guided Radiotherapy
One of the most exciting developments in preclinical radiotherapy research has been the introduction and increased adoption of small animal image-guided radiotherapy (SA-IGRT) platforms. Compared to traditional preclinical irradiation systems, which have rudimentary dose delivery capabilities with little or no treatment planning capabilities, SA-IGRT systems are designed with features that are routinely used in clinical radiotherapy: 3D imaging, inverse planning, and advanced dose delivery. Our group has several research programs developed around the SARRP system (Xstrahl Ltd). Two SARRP systems have been installed within our institution. One of them is dedicated to preclinical image-guided orthovoltage photon radiotherapy. The other has been integrated with our state-of-the-art proton therapy system at the Maryland Proton Treatment Center (MPTC) for preclinical image-guided proton therapy and FLASH research. The overarching goal is to translate preclinical findings into future clinical studies. Our major areas of research include:
- Development of tumor models in mice and rats for genitourinary, lung, and gastrointestinal cancers;
- Investigations of thermally modulated radiotherapy (hyperthermia) to sensitize tumor tissue while protecting surrounding normal tissue (thereby widening the therapeutic window);
- Investigations of advanced imaging techniques to spatially map postradiotherapy inflammation in lung stereotactic body radiotherapy;
- Exploration of the proton FLASH sparing effect in small animal models of human cancers.
Next Generation Motion Management
Accounting for temporal anatomic changes in thoracic and abdominal cancer, radiotherapy is one of the key scientific and clinical challenges of our era. These anatomic changes reduce image quality and target accuracy, which in turn leads to geometric and dosimetric errors in treatment. Such errors become increasingly important as we move toward hypofractionated regimens, such as stereotactic ablative radiotherapy, where highly potent doses are administered to the tumor target in just a few fractions. Thoracic anatomy changes in all four dimensions (4D = 3D + time) from cycle to cycle and day to day. A common limitation of current motion management techniques is that they discard large amounts of this 4D information and neither capture nor adequately account for cycle-to-cycle variations. The goal of our motion management research is to capture and account for all four dimensions at each of the three radiotherapy steps: simulation, treatment planning, and dose delivery. We use high-performance CPU and GPU computing to:
- Create patient-specific, parameterized volumetric motion models that describe the underlying patient anatomy as a function of optical surface over many breathing cycles;
- Investigate higher-order inverse planning strategies using GPU-based swarm optimization algorithms to create truly 4D-optimized treatment plans that use motion as an additional degree of freedom in the optimization process;
- Track real-time motion using a dynamic multileaf collimator that reshapes the beam to follow all of the complex changes (translation, rotation, and deformation) of the tumor and surrounding organs.
For more information about image guidance for precision small animal radiotherapy, please contact Kai Jiang, PhD, at KaiJiang@umm.edu.